7. Level issues
All analogue and acoustic inputs and outputs - or terminals - of an audio device can accept a certain maximum level input signal - above this level the device’s electronic circuit or mechanical construction connected to the terminal will clip. Also, the connected electronic circuits possess a noise floor that is independent from the signal level flowing through them.
As clipping of terminals cause unintended distortion, and noise floors limit the audio signal in the level dimension, system designers and sound engineers spend a considerable amount of their working time to manage the levels in audio systems to produce the highest dynamic range, and to make sure the signal never causes a terminal to clip. Also, signal chain levels must be constantly monitored and controlled to prevent clipping in case the SPL of the sound source or the output level of one of the system’s processes is higher than anticipated.
7.1 0dBFS
An audio system comprising of two or more audio devices possesses four or more terminals, each with their own clip level and noise floor. A signal in such a system passes all terminals on its route from the system input to the system output - risking to clip each terminal, and also picking up all noise levels. The ratio of the system’s lowest clip level and the accumulated noise level constitutes the system’s dynamic range or clip to noise ratio. There are three basic methods to route a signal through the successive devices in an audio system: random level, matched noise floors and matched clip levels - or 0dBFS - where FS stands for Full Scale: the highest level a terminal can handle without clipping. Figure 702 presents the three methods for a system of two devices, displaying the dynamic range of each terminal as a gray bar with the top representing the terminal’s clip level and the bottom representing the terminal’s noise floor. The resulting dynamic range is the ratio between the lowest clip level in the signal path and the accumulated noise floor at the last terminal.
All analogue and acoustic inputs and outputs - or terminals - of an audio device can accept a certain maximum level input signal - above this level the device’s electronic circuit or mechanical construction connected to the terminal will clip. Also, the connected electronic circuits possess a noise floor that is independent from the signal level flowing through them.
As clipping of terminals cause unintended distortion, and noise floors limit the audio signal in the level dimension, system designers and sound engineers spend a considerable amount of their working time to manage the levels in audio systems to produce the highest dynamic range, and to make sure the signal never causes a terminal to clip. Also, signal chain levels must be constantly monitored and controlled to prevent clipping in case the SPL of the sound source or the output level of one of the system’s processes is higher than anticipated.
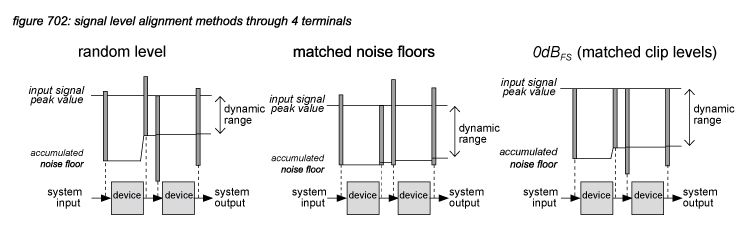
Figure 702 shows that when a random level alignment is used to route a signal through the audio system (which is any configuration other than matched noise floors or 0dBFS), the system’s dynamic range is the smallest because the dynamic range of individual terminals always overlaps with others. Matching noise floors minimises overlapping - resulting in a higher dynamic range, but it has the risk that the dynamic range of the last two devices in the signal path - the power amplifier and the speaker - are not always optimally used, causing higher costs. The 0dBFS method has the highest dynamic range because the noise floors are kept at the lowest level at each terminal - resulting in the lowest accumulated noise floor. Also, the 0dBFS method offers the lowest risk of clipping because all terminal clipping levels are aligned to the same level. Only the first terminal, which is normally the microphone’s acoustic input, can clip as a result of excessive input SPL - all other terminals can never clip (at unity gain). The conclusion is that the 0dBFS method is the optimal strategy to make the most use of a system’s dynamic range with the lowest risk of clipping and the lowest cost.
Assuming that the digital part of a networked audio system is always referenced to 0dBFS, there are five main parameters to align the system’s acoustical and analogue terminals: the selection of the microphones, power amplifiers and speakers, the setting of the analogue gain of the head amp, and the voltage gain of the power amplifier.
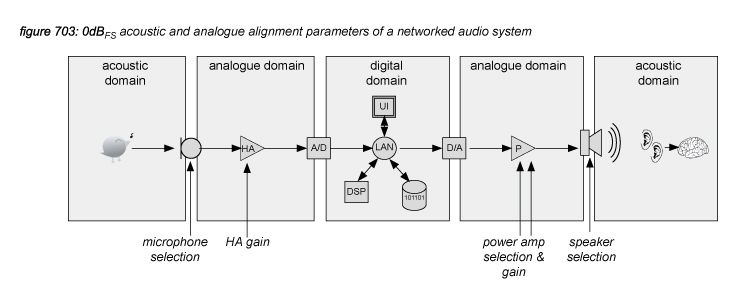
7.2 Head amps
As networked audio systems allow the placement of analogue inputs at remote locations - with the connection between the remote location and the control location (a mixing console) using an audio network protocol - the head amps that align the analogue microphone input levels with the remote input’s A/D converter is also located at a remote location. To control the head amp’s gain, high pass filter and phantom power, a remote head amp protocol must be used to control these functions from the control location. The head amp’s gain is most commonly a combination of relays and/or analogue switches that provide a variable gain in the analogue domain. A digital make-up can be added to increase the gain step accuracy and to provide constant gain to the network (see chapter 6.3).
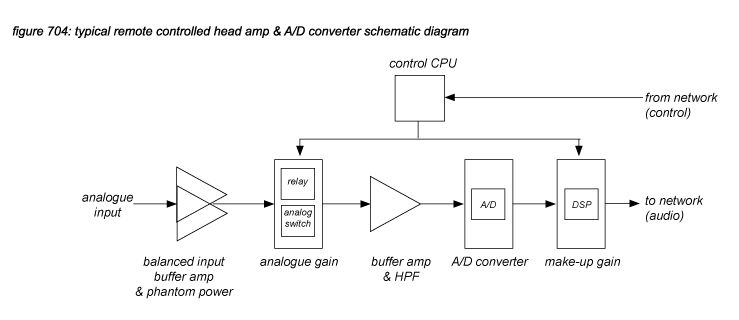
The noise floor and distortion of most high quality head amp circuits are normally very low. Designs from manufacturers with a natural sound philosophy provide a ‘flat’ transfer function from analogue input to A/D converter input - with as less as possible colouration, allowing DSP processes to apply sound colouration to the full control of the system designer and sound engineer. Some manufacturers apply a deliberate EQ curve in the analogue circuit to create a default Response that suits dedicated applications - eg. ‘British sound’ or ‘warm sound’.
To support a flexible use of networked audio systems, it is of vital importance that the gain step accuracy is high - so that when a preset is recalled on the control user interface (mixing console), the recalled gain is as accurate as possible. The human auditory system is capable of detecting level differences of less than one dB. Also, to provide a high consistency when loading presets in a different console of the same type, or when reconnecting a sound source to another input in the system, the gain consistency between channels and between input head amps in different stage boxes must be very high. Still, because the total gain between a system’s analogue input and an analogue output involves many electronic circuits, the accumulated signal chain gain consistency (or gain error) can be audible. This means that - even with very consistent head amps (and power amps) - every time a system is set up and connected, the head amp gains (and power amplifier gains) might have to be adjusted to comply to the design specifications.
7.3 Gain compensation
In networked audio systems where multiple DSP processes and user interfaces (eg. mixing consoles) share the same inputs (eg. stageboxes), head amp control becomes an issue. When one user interface changes the analogue gain of a head amp to suit its related DSP process, the signal level to all other DSP processes changes as well. If the operators of the other processes are unaware of the analogue gain change, this can cause troubles - so in general, if multiple processes use the same inputs, the operators of the processes (in case of mixing consoles the sound engineers) have to clearly communicate with each other to adjust digital gains in the DSP process manually to compensate for the analogue gain change in the head amp. Two kinds of gain compensation schemes can be used to automate this process: console gain compensation and constant gain.
Console gain compensation
Console gain compensation can be used in systems with two mixing consoles that are capable of handling the same HA control protocol as the stagebox. In most cases, one console is dedicated as the HA master console, the other as HA slave console. The master console controls the head amp in the stage box through a HA control protocol that flows through the same network as the audio. If the stagebox receives a gain change command (or a gain change is made locally), it executes it and sends a HA status back to the master console to update its analog gain display, and to the slave console to compensate the gain change using the slave console’s digital gain. The advantage of console gain compensation is that it can be used with any digital audio format and network protocol. The disadvantage is that only stageboxes and mixing consoles that can handle the same HA control protocol can be used, and only mixers that have gain compensation implemented in the software.
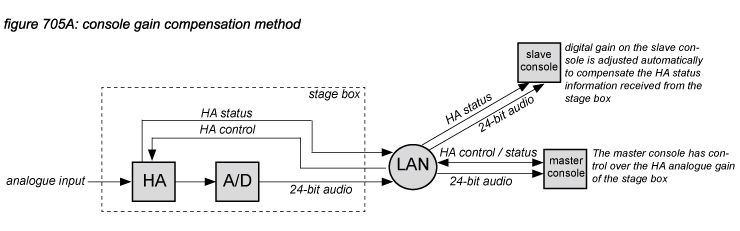
Constant gain
Constant gain can be used if the stagebox offers dedicated DSP to compensate the analogue gain in the digital domain before sending the audio data to the network. The advantage of constant gain systems is that any DSP process (mixer) can be used in any quantity, as the compensation happens in the stagebox and not in the receiving DSP process. In a constant gain system, multiple operators can control the gain, provided they support the HA control protocol used in the stagebox. A disadvantage of constant gain is that it requires a distribution network with a higher bit rate than the audio signal to provide full resolution, eg. a 32-bit network to support constant gain for 24-bit signals.
Using a constant gain system requires a more strict understanding of the difference between digital and analogue gain functionality. In conventional digital mixing consoles, although a digital gain is always provided, the analogue gain is often used to align the analogue input level for optimal A/D conversion, as well as to set the incoming level to the suit the mixing process. In a constant gain system, the analogue gain can be used exclusively for optimization of A/D conversion (to optimise audio quality), leaving each console’s digital gain to set the incoming level. More details on constant gain implications on audio quality is presented in chapter 6.3: Constant gain A/D converters.
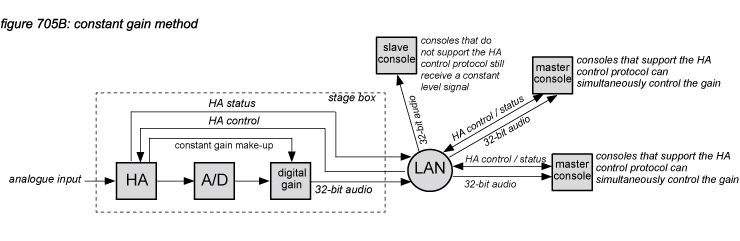
7.4 Clip level mismatch
If analogue devices are used before or after a networked audio system’s analogue inputs and outputs, such as external analogue pre-amplifiers or power amplifiers, then the corresponding terminals must be connected. If the clip levels of these terminals don’t match, the level difference can reduce the system’s dynamic range (in other words: waste it). As most analogue devices use buffer amp circuits to provide inputs and outputs that are independent of the connected terminal’s impedance, clip levels are a fixed value - they can not be changed by the system designer or sound engineer without opening the device cabinet to perform a hardware modification.
To illustrate how clip level mismatches cause the dynamic range to be decreased, figure 706 a, b and c present the connection of the analogue input of three different power amplifiers to a Yamaha DME24N analogue output, with different clip levels of the power amplifiers input as listed in table 701.
table 701: clip levels of the Yamaha DME24N and three different power amplifiers
device
terminal
clip level
Yamaha DME24N
output
+24 dBu
power amplifier P1
input
+24 dBu
power amplifier P2
input
+27 dBu
power amplifier P3
input
+21 dBu
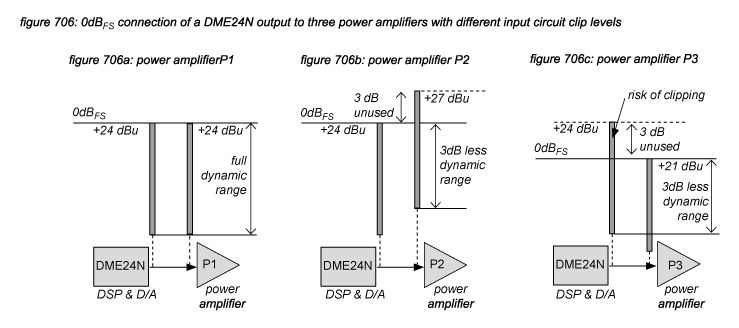
Clip level mismatches cause a system’s dynamic range to be limited by as much as several dB’s - raising the system’s noise floor by several dB’s. Specially for systems with high power loudspeakers this can be a problem - as the noise floor below 100dBFS becomes audible for the audience situated close to the speakers. If the mismatch causes a clipping risk - as in figure 706c where the power amplifier clip level is lower than the system output’s clip level - the system designer or sound engineer has to manually limit the system’s output level in the digital domain.
In every networked audio system design, it is advised to check the clip level specifications at every line input (eg. wireless receivers) and output (eg. power amplifiers) of the system to know if any digital gain should be designed into the DSP processes to prevent mismatch clipping. Some devices offer internal DIP switches or jumpers with a choice of multiple clipping values. For high power high quality applications, it might be worth while to consider an external passive analogue attenuator if an internal option is not available.
7.5 Double A/D-D/A pass signal paths
In digital audio systems, the A/D and D/A converters and their analogue circuits are the main contributors to the system’s noise floor. It makes sense for system designers to make sure that all signal paths flow through only one A/D converter and one D/A converter. If signal paths flow through such an A/D-D/A pass twice, the peak value of the noise floor is doubled, and the RMS level raises with several dB’s. In the migration from analogue to digital live mixing consoles in the past 2 decades, often the connections to the speaker processing equipment remained analogue - even when the analogue processors were replaced by digital versions. In such a case, often the system’s dynamic range can be improved by several dB’s by simply changing the connection between the mixing console and the speaker processors from analogue to digital.
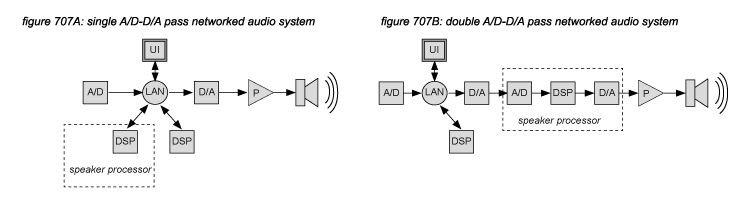
7.6 Unbalanced output mode
Output compression is sometimes applied in speaker processing for multi-amped speaker cabinets to protect drivers from overloading where other drivers in the same cabinet can still output more SPL. Also, full range output compression is sometimes applied to multi-cabinet speaker systems where parts of the system have spare SPL headroom. In both cases, the compression is used to make the system ‘louder’ - leaving out frequency ranges or locations above a certain threshold level. This could be seen as an audio quality problem - affecting a speaker system’s Performance. But because it is applied as an intended process - with the goal to make the system louder at the cost of losing the designed SPL balance between loudspeakers at high volumes, it affects the Response (sound quality) - and not the Performance (audio quality).
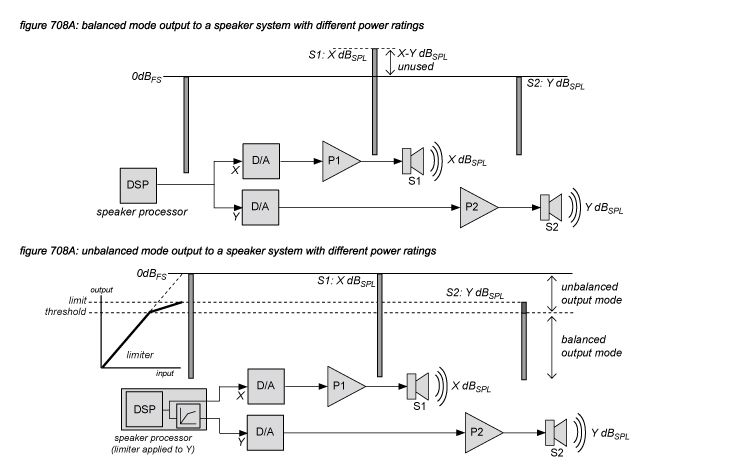